Introduction
A carbonyl compound is a molecule containing functional group that consists of a carbon atom double-bonded to an oxygen atom (C=O). It’s like the dynamic duo of organic chemistry, showing up in various compounds. These compounds play a starring role in the organic world, and their primary members include aldehydes, ketones, and carboxylic acids. Carbonyl compounds also include other cool characters like esters, amides, and lactones. They’re like the supporting cast, each with its unique role. Carbonyl compounds are the VIPs of organic chemistry, strutting their stuff with that C=O swagger. In this article we will see aldehydes, ketones, their reactions and some important compounds of the same.
Aldehydes
Aldehydes belong to a class of organic compounds characterized by a carbonyl group (―C=O). This carbonyl group consists of a carbon atom double-bonded to an oxygen atom. The general structural formula for an aldehyde is R-CHO, where R represents either a hydrogen atom or a hydrocarbon group (alkyl or aryl) attached to the carbonyl carbon.
Bonding and Reactivity
- The carbon-oxygen double bond in aldehydes is significant. Oxygen is more electronegative than carbon, so it pulls electrons toward itself.
- Aldehydes have a hydrogen atom directly attached to the carbonyl carbon. This unique arrangement affects their reactivity.
- Oxidation of aldehydes yields acids (think of it as adding an oxygen), while reduction produces alcohols (removing that oxygen).
Physical Properties
- Solubility: Smaller aldehydes are more soluble in water. Formaldehyde (HCHO) and acetaldehyde (CH₃CHO) are completely soluble.
- Odor: Many aldehydes have characteristic odors. For example, formaldehyde has a pungent smell.
- Boiling Points: Aldehydes generally have lower boiling points than corresponding alcohols or carboxylic acids due to their smaller size and lack of extensive hydrogen bonding.
Examples
- Methanal (Formaldehyde): Used in embalming fluids and as a disinfectant.
- Ethanal (Acetaldehyde): Found in alcoholic beverages and used in the production of chemicals.
- Propanal (Propionaldehyde): Used in the synthesis of pharmaceuticals and perfumes.
Ketones
Ketones are carbonyl compounds that contain a carbon-oxygen double bond (C=O). Unlike aldehydes, which have a hydrogen atom directly attached to the carbonyl carbon, ketones have two hydrocarbon groups flanking the carbonyl group.
Structure and Naming
The general formula for a ketone is R-CO-R’, where R and R’ represent alkyl or aryl groups. For example, propanone (commonly known as acetone) is written as CH₃COCH₃.
Physical Properties
- Boiling Points: Ketones have higher boiling points than alkenes and ethers but lower than alcohols of similar molecular weight. Why? Well, ketones can’t donate hydrogen for hydrogen bonding (they lack hydrogen atoms directly attached to the carbonyl group), but their polarity still gives them a boost.
- As the ketone molecule size increases, so does its boiling point.
- Solubility: Ketones are highly polar and moderately soluble in water due to their carbonyl group. Smaller ketones (like acetone) are completely miscible with water.
- Volatility: Ketones are quite volatile—think of that familiar smell when you open a bottle of nail polish remover.
Reactivity
- Ketones are relatively stable and resist oxidation. Unlike aldehydes, they don’t readily undergo further oxidation to form carboxylic acids.
- They participate in nucleophilic addition reactions at the carbonyl carbon, making them versatile in organic synthesis.
Examples
- Acetone (Propanone): Found in nail polish removers and industrial solvents.
- Butanone (Methyl ethyl ketone, MEK): Used in paint thinners and adhesives.
- Cyclohexanone: An important intermediate in nylon production.
Nucleophilic addition
To learn nucleophilic addition, we must know what is nucleophile. Nucleophiles are electron-rich species—like friendly atoms with extra electrons. When a nucleophile encounters a carbonyl group, it’s like a chemistry waltz. The nucleophile gracefully attacks the carbonyl carbon (the positively charged one) and forms a new bond. Due to this attack A C-Nu bond (where Nu represents the nucleophile), and the original C=O pi bond breaks. The carbon’s geometry changes from trigonal planar to tetrahedral. After this addition, the oxygen carries a negative formal charge.
Examples
Cyanohydrins: Picture a cyanide ion (CN⁻) elegantly waltzing in. It forms a new sigma bond with the carbonyl carbon, breaking the C=O double bond and forms cyanohydrin.
Hydration of Aldehydes and Ketones: Here’s an acid-catalyzed dance. Protonation activates the carbonyl, and water (our nucleophile) gracefully attacks the carbonyl group, forming an alcohol derivative.
Why Do Carbonyl Compounds Love Nucleophilic Addition?
- In carbonyl compounds, the carbon-oxygen bond is polar. Oxygen’s electronegativity creates higher electron density near itself, leading to a partial negative charge on the oxygen atom and a partial positive charge on the carbon atom.
- The carbonyl carbon, with its partial positive charge, behaves as an electrophile—a chemistry flirt, if you will.
- To stabilize the partial negative charge on the oxygen, we sometimes introduce an acidic group. The proton from the acid bonds with the carbonyl oxygen, neutralizing the negativity.
Electromeric Effect
The Electromeric Effect happens in organic compounds with multiple bonds (like double or triple bonds). When an attacking reagent shows up, the electrons involved in these multiple bonds decide to shift their position. One moment they’re shared, and the next, they’re fully transferred to one of the atoms. Due to this shift in position, the molecule becomes temporarily polar—a bit like a magnet with a positive and negative end.
But remember, this effect is like a fleeting cameo—it only lasts while the attacking reagent is around. Once it leaves, the molecule goes back to its original state.
Types of Electromeric Effects
- Positive Electromeric Effect: In this case, the pi electrons (those involved in the multiple bond) move toward the attacking reagent. It’s like sharing your lunch with a friend. This happens when the attacking reagent is an electrophile (loves electrons) and bonds with the atom that got the extra electrons.
- Negative Electromeric Effects: Here, the pi electrons move away from the attacking reagent. It’s like saying, “Nope, you can’t have my electrons!” The attacking reagent bonds with the positively charged atom (the one that lost the electrons). This effect happens with nucleophiles (electron huggers).
Examples
+E Effect: Picture an aldehyde (you know, those carbonyl compounds with a snazzy C=O group). When an electrophile (like an acid) approaches, the pi electrons scoot toward the electrophile. Protonation of ethene (good ol’ C₂H₄) is a classic example.
-E Effect: Now, let’s talk carbonyl compounds (aldehydes and ketones). When a nucleophile (like a chemistry hugger) comes knocking, the pi electrons move away from the nucleophile. Result? Nucleophilic addition! For instance, when a cyanide ion (CN⁻) cozies up to an aldehyde, those pi electrons head toward the oxygen atom, creating a new bond.
Aldol Condensation
An aldol condensation is a chemical reaction that involves two key steps:
Aldol Reaction (Step 1)
- Imagine two carbonyl compounds—either aldehydes or ketones—hanging out. These carbonyl compounds have a carbon-oxygen double bond (C=O).
- When they meet, they decide to do a little chemistry dance. One of them (let’s call it Compound A) donates its hydrogen atom to the other (Compound B).
- As a result, Compound B gains a new carbon-carbon bond, forming a β-hydroxyaldehyde or a β-hydroxyketone. We call this combined product an aldol (a fusion of “ald” from aldehyde and “ol” from alcohol).
- So, in simple terms, the aldol reaction creates a new bond between two carbonyl compounds, resulting in an aldol.
Dehydration (Step 2)
- Now that we have our aldol, it’s time for the next act. The aldol undergoes dehydration.
- Dehydration means removing a water molecule (H₂O). In our case, the water comes from the aldol.
- As a result, The aldol loses a water molecule, and the remaining structure becomes a conjugated enone (a fancy term for a molecule with alternating double bonds).
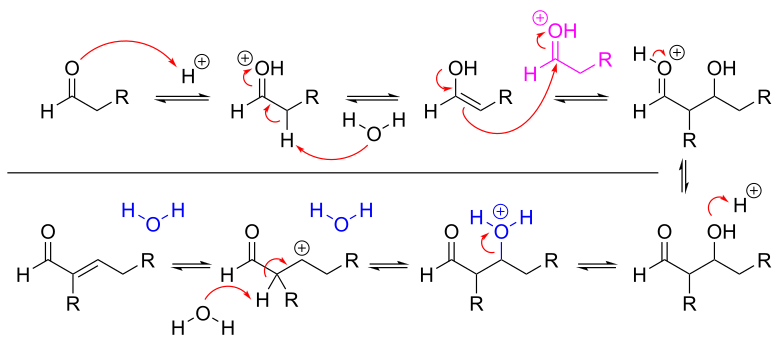
Why Is It Important
- Aldol condensations are crucial in organic synthesis because they allow us to form new carbon-carbon bonds. These bonds are like the building blocks of complex molecules.
- For example, the Robinson annulation reaction sequence features an aldol condensation. The product, known as the Wieland-Miescher ketone, becomes a starting material for various organic syntheses.
Example
Imagine two aldehydes—one with the formula CH₃CHO and the other with the formula CH₃C(O)CH₂CHO.
They undergo an aldol reaction, resulting in a β-hydroxyaldehyde:
CH₃CHO + CH₃C(O)CH₂CHO → CH₃CH(OH)CH₂C(O)CH₂CHO (the aldol)
Next, dehydration occurs, and the water molecule is removed:
CH₃CH(OH)CH₂C(O)CH₂CHO → CH₃CH=CHC(O)CH₂CHO (the conjugated enone).
Crossed Aldol Condensation
Imagine two different carbonyl compounds (either aldehydes or ketones) hanging out in a protic solvent (like water or alcohol). They decide to do a chemical tango—a condensation dance. Each carbonyl compound can act as both a dancer and a partner. As a result, We get a mixture of products—four of them, to be precise. But here’s the catch: this mixture isn’t very useful for synthesis.
Why Four Products?
- Both carbonyl compounds have alpha hydrogens (those attached to the carbon next to the carbonyl group).
- So, they can form carbanions (like chemistry ninjas with extra electrons) and also accept carbanions.
- When they dance together, they exchange partners. It’s like a wild swing dance at the molecular level!
- The outcome: Four different products, but unfortunately, they don’t lead us to anything particularly valuable.
When Does Crossed Aldol Happen
- It occurs between two distinct aldehydes or ketones.
- If both aldehydes have alpha hydrogens, they can play both roles—donor and acceptor.
- But if one of them lacks alpha hydrogens, it can only accept the carbanion.
- The result: Either a mixture of four products (when both have alpha hydrogens) or just two products (when one lacks alpha hydrogens).
Example: Benzaldehyde and Acetophenone
- Picture benzaldehyde (no alpha hydrogen) and acetophenone (has alpha hydrogen) hitting the dance floor.
- They undergo crossed aldol condensation in the presence of dilute NaOH.
- As a result, A β-hydroxy ketone forms.
- And guess what? Dehydration quickly follows, creating an α, β-unsaturated ketone.
Cannizzaro Reaction
The Cannizzaro reaction is named after the Italian chemist Stanislao Cannizzaro (1853). It’s a fascinating redox reaction that involves aldehydes. Specifically, it occurs when two molecules of a non-enolizable aldehyde (an aldehyde without alpha-hydrogens) react in the presence of a concentrated alkali (like sodium hydroxide, NaOH). As a result, one aldehyde molecule gets oxidized to form a carboxylic acid, while the other gets reduced to yield a primary alcohol.
Mechanism
- Step 1: A hydroxide ion (OH⁻) acts as a nucleophile and attacks the carbonyl group of one aldehyde. This leads to a disproportionation reaction, creating an anion with two negative charges.
- Step 2: The resulting intermediate now behaves as a hydride reducing agent. It releases a hydride ion (H⁻), which goes on to attack another aldehyde molecule. The doubly charged anion becomes a carboxylate anion, and the aldehyde transforms into an alkoxide anion.
- Step 3: Water steps in and donates a proton to the alkoxide anion, forming the final alcohol product. Why does this work? Because the alkoxide is more basic than water. Meanwhile, the carboxylate ion, less basic than water, gives rise to the final carboxylic acid product when acid workup is used.
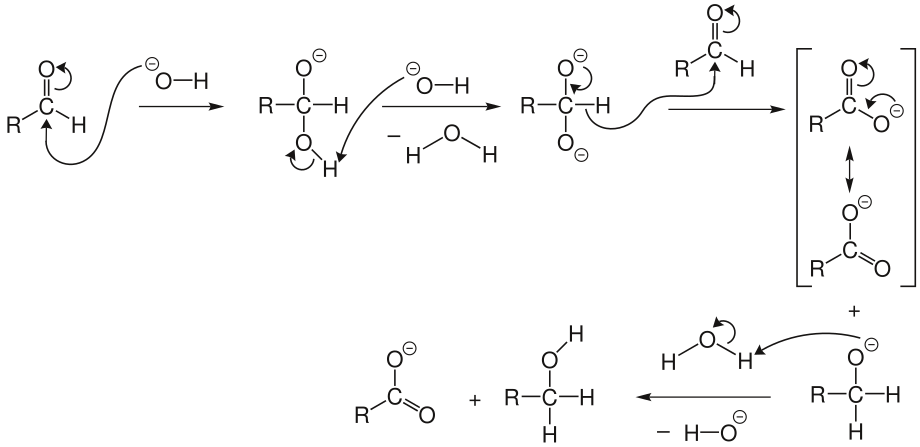
Crossed Cannizzaro Reaction
Sometimes, we want better yields. That’s where the crossed Cannizzaro reaction comes in. Instead of using two identical aldehydes, we combine a sacrificial aldehyde with a more valuable chemical. Formaldehyde often plays the role of the reductant, oxidizing it to sodium formate. The required alcohol is then obtained from the reduction of the other aldehyde. As a result, Efficiency is improved.
Why “Unwanted Byproducts”?
In basic conditions, the Cannizzaro reaction can sometimes produce side products we don’t want. These byproducts arise due to the complexity of aldehyde reactions in strong bases.
Benzoin Condensation
The Benzoin condensation is a chemical reaction that involves two molecules of benzaldehyde (an aromatic aldehyde) coming together. In this reaction, they join forces to form a compound called benzoin. The key players here are benzaldehyde molecules, and they perform a molecular handshake to create something new.
Mechanism
- Imagine two benzaldehyde molecules in a room. They decide to dance (chemically, of course!).
- First, a cyanide ion (CN⁻) joins the party. Cyanide is like the matchmaker—it helps the reaction along.
- The cyanide ion reacts with one benzaldehyde, forming a cyanohydrin intermediate. This intermediate is stable and ready for the next step.
- Now, the cyanohydrin intermediate meets another benzaldehyde. They hold hands (well, sort of) and undergo a condensation reaction.
- The result? Benzoin! It’s like a ketone with a twist—acetophenone with hydroxy and phenyl groups at the alpha-position (α‐hydroxyl ketone).
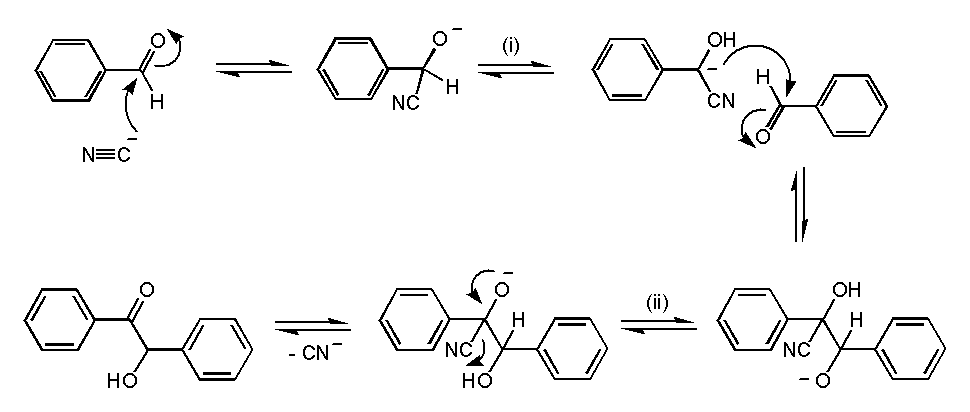
Applications
- Polymer Hardening: Benzoin plays a role in hardening various polymers. It’s like adding strength to their molecular structure.
- Heterocyclic Synthesis: Chemists use benzoin to create complex ring structures (heterocycles). Think of it as building intricate molecular puzzles.
- Aliphatic Aldehydes: Benzoin’s influence extends beyond the aromatic world. It also works with aliphatic aldehydes (those without the aromatic ring).
Remember vitamin B1? That’s thiamine! It can also catalyze the benzoin condensation.
Thiamine + sodium hydroxide = free thiamine. And free thiamine can initiate this reaction.
Perkin Condensation
- The Perkin condensation (also known as the Perkin reaction) is an organic reaction used to synthesize α,β-unsaturated aromatic acids.
- Here’s the setup: An aromatic aldehyde (like benzaldehyde) and an acid anhydride (with at least two α-hydrogen atoms) come together for a chemical tango.
- The dance floor includes an alkali salt of the acid (acting as a weak base).
- The result? We get a new compound—an α,β-unsaturated aromatic acid.
Example: Benzaldehyde to Cinnamic Acid
- Imagine benzaldehyde (our aromatic aldehyde) joining forces with an acid anhydride.
- In the presence of an alkali salt, they condense and form cinnamic acid.
- Cinnamic acid has that delightful α,β-unsaturated bond in its structure.

Mechanism
The mechanism involves several moves:
- Formation of anhydride enolates and aldol-type condensation leads to an alkoxide anhydride.
- Intermolecular acylation creates an acetoxy carboxylate, which then forms a mixed anhydride.
- Elimination of acetic acid and subsequent hydrolysis give us the unsaturated acid (our cinnamic acid).
Summary
Carbonyl compounds (aldehydes and ketones) engage in nucleophilic addition, creating new bonds. The Electromeric Effect involves electron shifts, while aldol condensation leads to β-hydroxyaldehydes or β-hydroxyketones. Crossed aldol condensation mixes different carbonyl compounds. The Cannizzaro reaction balances oxidation and reduction. Benzoin condensation forms α,β-unsaturated compounds, and Perkin condensation yields aromatic acids.
For more regular updates you can visit our social media accounts,
Instagram: Follow us
Facebook: Follow us
WhatsApp: Join us
Telegram: Join us
1 thought on “Carbonyl Compound- Reactions”