Citric acid cycle (Krebs cycle)
The citric acid cycle, also known as the Krebs cycle, is another key player in the energy production game. It takes over from glycolysis, grabbing the pyruvate from that process. Imagine it as the main course after the glycolysis appetizer. Here, the citric acid cycle breaks down pyruvate further, squeezing out even more energy through a series of reactions. Unlike glycolysis, it relies on oxygen and works in the cell’s powerhouse, the mitochondria. This cycle generates ATP, the cellular energy currency, and also produces important energy carrier molecules. It’s a complex dance, but ultimately fuels many cellular functions. In this article we will see pathway, energetics and significance of Krebs cycle.
Pathway
The Krebs cycle, also known as the citric acid cycle or TCA cycle, is a central metabolic pathway happening within the mitochondrial matrix of eukaryotic cells. It acts as the main stage for extracting energy from carbohydrates, fats, and proteins. Here’s a breakdown of the pathway:
- Acetyl CoA Enters the Cycle: The cycle begins with a 2-carbon molecule called acetyl CoA, derived from the breakdown of pyruvate in glycolysis. Acetyl CoA donates its acetyl group to a 4-carbon molecule called oxaloacetate, forming a new 6-carbon molecule called citrate.
- A Series of Transformations: Citrate undergoes a series of eight enzymatic reactions, each transforming the molecule and releasing energy. These reactions can be broadly categorized as:
- Decarboxylation: Removal of a carbon dioxide molecule (CO2)
- Dehydrogenation: Removal of hydrogen atoms, capturing the energy in the form of NADH and FADH2 (important electron carrier molecules)
- Substrate-level phosphorylation: Direct transfer of a phosphate group to ADP, generating ATP
- Key Products
- Carbon Dioxide: Two CO2 molecules are released throughout the cycle, representing the breakdown of the original pyruvate molecule.
- ATP: A net gain of 3 ATP molecules is generated through substrate-level phosphorylation.
- NADH and FADH2: These energy carriers capture a significant amount of energy removed during dehydrogenation reactions. They will later donate their electrons to the electron transport chain (ETC) for even more ATP production.
- Regeneration of Oxaloacetate: At the end of the cycle, the molecule is regenerated back to oxaloacetate, ready to accept another acetyl CoA and continue the cycle. This ensures a continuous process for energy extraction.
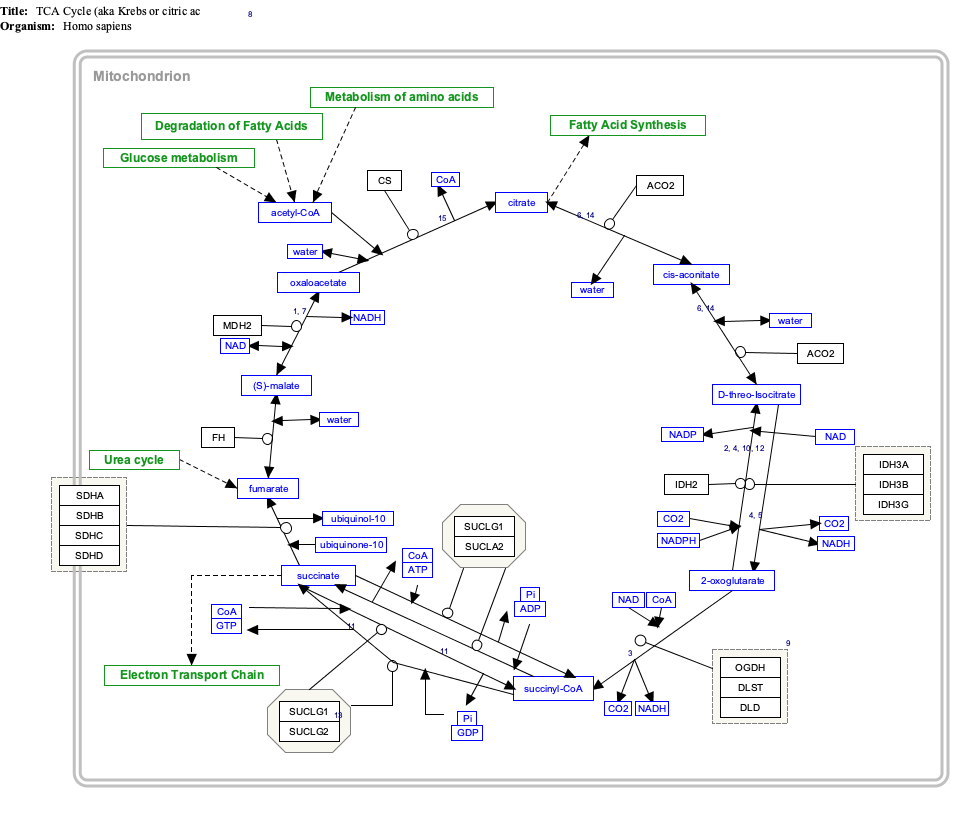
Energetics
The Krebs cycle, while not the biggest ATP producer itself, plays a vital role in setting the stage for significant energy generation. Here’s a breakdown of the energetics:
Inputs
- 1 molecule of Acetyl CoA (derived from pyruvate)
- 3 molecules of NAD+
- 1 molecule of FAD
- 4 molecules of ADP
- 4 molecules of inorganic phosphate (Pi)
Outputs
- 3 molecules of NADH
- 1 molecule of FADH2
- 1 molecule of GTP (can be converted to ATP)
- 2 molecules of CO2
- Coenzyme A (CoA)
Energy Production
- Direct ATP production: The Krebs cycle directly generates 1 molecule of ATP through substrate-level phosphorylation.
- Indirect ATP production: The key lies in the generated NADH and FADH2 molecules. These capture energy during the cycle and act as electron carriers.
Later, in the electron transport chain (ETC), they donate their electrons to a series of protein complexes, ultimately leading to the production of a significant amount of ATP (around 30-34 ATP per NADH and 2 ATP per FADH2).
Net Gain
While the Krebs cycle directly generates only 1 ATP, the captured energy in NADH and FADH2 has the potential to produce much more ATP through the ETC.
Here’s a simplified breakdown
- Direct ATP: 1
- Potential ATP from NADH (3 molecules): 90-102 ATP (depending on the efficiency of the ETC)
- Potential ATP from FADH2 (1 molecule): 2 ATP
Therefore, the total ATP yield from the Krebs cycle, including its contribution to the ETC, can be around 93-104 ATP per molecule of glucose (considering 2 acetyl CoA molecules are generated from 1 glucose).
Additional Points
- GTP can be converted to ATP within the cell, further adding to the overall energy gain.
- The cycle is designed for efficiency. The CoA released allows for the continuous breakdown of more Acetyl CoA molecules.
The Krebs cycle, along with the ETC, forms a powerful duo for maximizing energy extraction from food molecules.
The Significance of the Krebs Cycle
- Efficient Energy Production: The Krebs cycle extracts significantly more energy from glucose compared to glycolysis. It utilizes the captured energy in NADH and FADH2 to generate even more ATP in the subsequent electron transport chain.
- Metabolic Hub: The cycle acts as a central hub for various metabolic pathways. Acetyl CoA, derived from fats and proteins, can also enter the Krebs cycle, allowing these molecules to be broken down for energy production.
- Regulation: The Krebs cycle is tightly regulated based on the cell’s energy demands. The availability of substrates (acetyl CoA) and oxygen influence the rate of the cycle.
Overall, the Krebs cycle is a crucial pathway that efficiently extracts energy from various food sources, laying the foundation for cellular energy production.
Summary
The Krebs cycle, a key player in cellular energy production, takes center stage within the mitochondria. It utilizes a 2-carbon molecule (acetyl CoA) derived from glucose breakdown to fuel a series of eight enzymatic reactions. These reactions generate ATP directly, remove carbon dioxide as waste, and capture significant energy in NADH and FADH2 molecules. Unlike glycolysis, the Krebs cycle operates in an oxygen-dependent environment and acts as a metabolic hub, processing products from fat and protein breakdown as well. This efficient cycle, tightly regulated based on cellular needs, lays the foundation for further energy production through the electron transport chain, ultimately powering various cellular functions.
For more regular updates you can visit our social media accounts,
Instagram: Follow us
Facebook: Follow us
WhatsApp: Join us
Telegram: Join us