Nervous system
The nervous system- At the core of every action, thought, and sensation lies the most complex and enigmatic network within the human body. A symphony of electrical impulses, it orchestrates the delicate dance between mind and matter, weaving the fabric of consciousness and control. This biological marvel not only connects the intricate pathways from the brain to the furthest reaches of the body but also governs the silent yet vital conversations between neurons. As we embark on this exploration, we delve into the depths of the central and peripheral nervous systems, uncovering the mysteries of neural communication and the profound impact it has on our existence. Join us as we journey through the cerebral corridors and neural networks that define our interactions with the world and ourselves.
Organisation of nervous system
The nervous system is a complex, highly organized network that coordinates all the activities of the body. It is divided into two main parts: the Central Nervous System (CNS) and the Peripheral Nervous System (PNS).
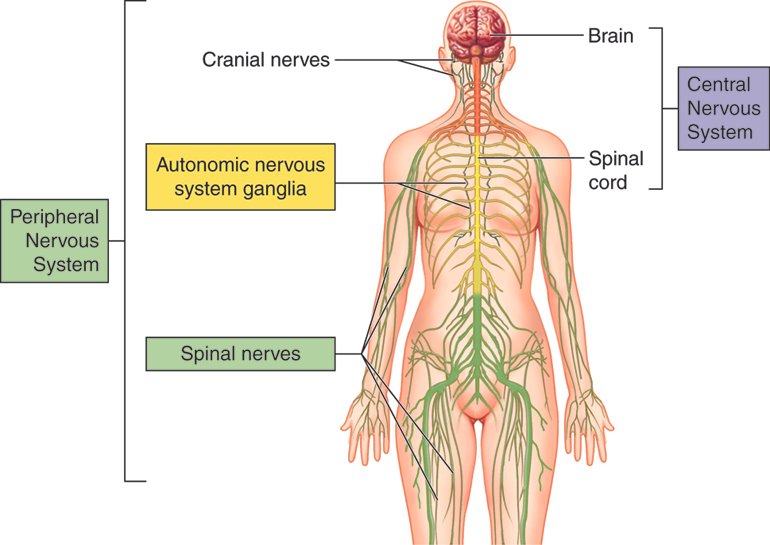
Central Nervous System (CNS)
The CNS is the command center of the body and is composed of the brain and the spinal cord.
- The brain is the control center for thought, memory, emotion, touch, motor skills, vision, breathing, temperature, hunger, and every process that regulates our body.
- The spinal cord is the major information pathway between the brain and the peripheral nervous system.
Peripheral Nervous System (PNS)
- The PNS consists of all the neural tissue outside the CNS.
- It is divided into the somatic nervous system and the autonomic nervous system.
- The somatic nervous system controls voluntary movements and transmits sensory information to the CNS.
- The autonomic nervous system controls involuntary body functions and is further divided into the sympathetic and parasympathetic nervous systems.
Neurons and Glial Cells
- Neurons are the basic working units of the nervous system, designed to transmit information to other nerve cells, muscle, or gland cells.
- They have a cell body, dendrites, and an axon. The axon carries signals away from the cell body, and dendrites bring information to the cell body.
- Glial cells provide support and protection for neurons; they are more numerous than neurons and are found throughout the nervous system.
Gray Matter and White Matter
- Gray matter consists of neuron cell bodies and is found in the brain and spinal cord.
- White matter consists of myelinated axons and is responsible for communication between different areas of the CNS and between the CNS and the rest of the body.
Functional Divisions
- The nervous system can also be divided functionally into the afferent and efferent
- The afferent system transmits sensory information from the body to the CNS.
- The efferent system carries commands from the CNS to the muscles and glands.
Neuron
A neuron, also known as a nerve cell, is the fundamental unit of the nervous system responsible for transmitting information throughout the body
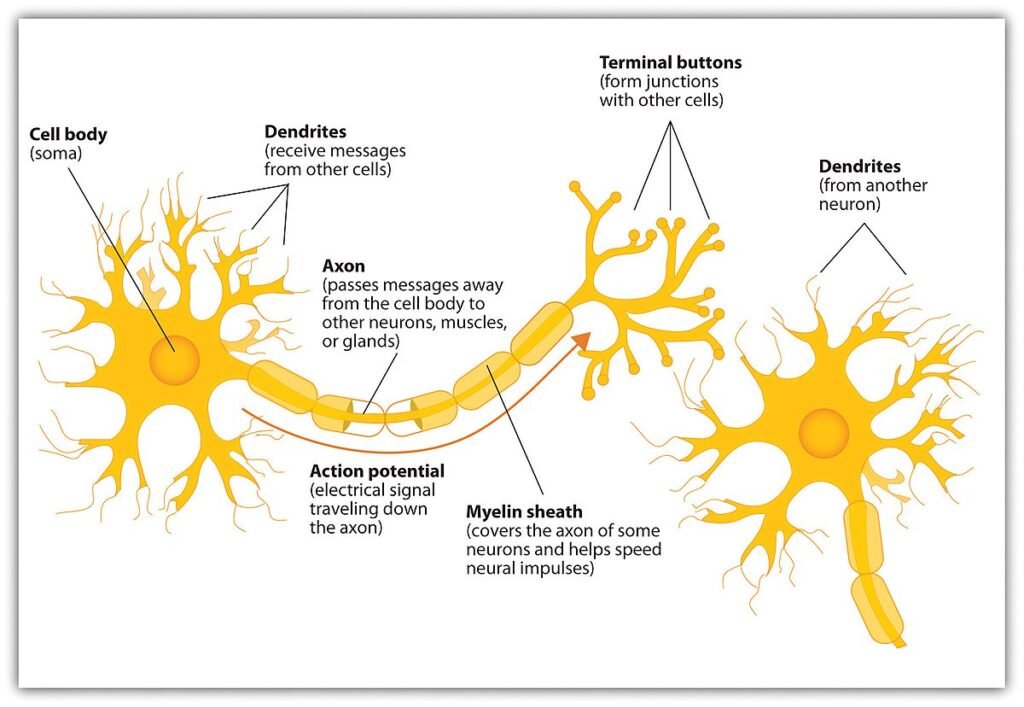
Structure of a Neuron
Cell Body (Soma): The cell body is the central part of the neuron that contains the nucleus, which houses the cell’s genetic material. The soma is responsible for maintaining the life of the neuron and also plays a role in analyzing the signals received.
Dendrites: These are tree-like extensions from the cell body that receive chemical signals from other neurons. The surface of dendrites is covered with synapses, which are the points of contact with other neurons.
Axon: This is a long, slender projection that conducts electrical impulses away from the cell body. Axons can vary in length, with some extending a few millimeters and others up to a meter or more in humans.
Myelin Sheath: Many axons are covered by this insulating layer, which is composed of a substance called myelin. The myelin sheath increases the speed at which impulses propagate along the axon.
Nodes of Ranvier: These are gaps in the myelin sheath along the axon. They are important for the rapid conduction of nerve impulses, allowing the impulse to “jump” from node to node.
Axon Terminals: The axon ends in a series of small swellings called axon terminals or terminal buttons. These contain synaptic vesicles filled with neurotransmitters, which are chemicals that can transmit the signal to the next neuron.
Function of a Neuron
- Transmission of Signals: Neurons communicate with each other through electrical impulses known as action potentials. These impulses travel down the axon to the axon terminals.
- Synaptic Transmission: When the action potential reaches the axon terminals, it triggers the release of neurotransmitters into the synaptic cleft, the small gap between neurons.
- Reception of Signals: The neurotransmitters cross the synaptic cleft and bind to receptors on the dendrites of the neighboring neuron, generating a new action potential in that neuron.
Types of Neurons
- Sensory Neurons: These neurons carry signals from sensory receptors to the CNS.
- Motor Neurons: They transmit commands from the CNS to muscles and glands.
- Interneurons: Found only in the CNS, they connect sensory and motor neurons and carry out complex processing.
Neurons are incredibly specialized and diverse, with different types and shapes adapted to their specific functions within the nervous system. The human brain alone contains approximately 86 billion neurons, each forming thousands of synaptic connections, making the network of neural communication extraordinarily complex and efficient.
Neuroglia
Neuroglia, often referred to as glial cells, are the non-neuronal cells in the nervous system that provide support and protection for neurons. They are essential for the proper functioning of the nervous system, performing a variety of tasks that help maintain homeostasis within the neural environment.
Types of Neuroglia
Astrocytes: These star-shaped cells are the most abundant glial cells in the CNS. They have many functions, including:
- Supporting and bracing neurons.
- Regulating the transmission of electrical impulses.
- Maintaining the blood-brain barrier, which controls the passage of substances from the bloodstream into the brain.
- Repairing and scarring processes in the brain following traumatic injuries.
Oligodendrocytes: These cells produce the myelin sheath in the CNS, which insulates neuronal axons to increase the speed of electrical transmission. Each oligodendrocyte can extend its processes to 50 axons, wrapping them with a myelin sheath.
Schwann Cells: Similar to oligodendrocytes but found in the PNS, Schwann cells also produce myelin. They are involved in the repair process after injury.
Microglia: These are the resident macrophages of the CNS, responsible for phagocytosis, which is the process of engulfing and absorbing pathogens and dead cell debris. They are also involved in immune defense in the brain.
Ependymal Cells: Lining the ventricles of the brain and the central canal of the spinal cord, ependymal cells are involved in the production and circulation of cerebrospinal fluid (CSF).
Satellite Cells: These are found in the PNS and have similar functions to astrocytes, providing support and nutrients to the surrounding neurons.
Functions of Neuroglia
- structural Support: Neuroglia provide a scaffold for neurons, helping to hold them in place.
- Myelination: Certain glial cells (oligodendrocytes and Schwann cells) create the myelin sheath around axons, which is critical for rapid signal transmission.
- Regulation of Ion Balance: Glial cells help regulate the ionic balance and concentration of neurotransmitters around neurons.
- Blood-Brain Barrier: Astrocytes are key in maintaining the integrity of the blood-brain barrier, which protects the brain from harmful substances in the blood.
- Injury Repair: Following injury, glial cells are involved in the brain’s healing process.
- Neurotransmitter Clearance: Glial cells clear excess neurotransmitters from the synaptic cleft to terminate the signal between neurons.
Recent Research on Neuroglia
- Recent studies have shown that neuroglia play a significant role in synapse formation, maintenance, and modulation. They are not just passive support cells but are actively involved in the cognitive functions of the brain by forming and maintaining synaptic circuits.
- Neuroglia out number neurons in the brain and are crucial for the health and functionality of the nervous system. Their contributions go beyond mere support, influencing neuronal function and overall brain activity.
Nerve fiber
A nerve fiber, also known as an axon, is a crucial component of a neuron, the cell that makes up the nervous system.
Structure of Nerve Fibers
- Axon: The main structure of a nerve fiber is the axon, which is a long, slender projection that extends from the neuron’s cell body.
- Myelin Sheath: Many axons are covered by a myelin sheath, which is a layer of fatty substance that helps to insulate the axon and increase the speed of electrical signal transmission.
- Nodes of Ranvier: These are gaps in the myelin sheath that facilitate the rapid conduction of nerve impulses along the axon.
- Axon Terminals: The ends of the axon, where the nerve fiber makes synaptic contacts with other neurons or effector cells.
Supporting Structures
- Endoneurium: Each individual nerve fiber is surrounded by a layer of connective tissue called the endoneurium.
- Perineurium: Groups of fibers are bundled together by another connective tissue layer called the perineurium.
- Epineurium: The entire nerve, which is a bundle of many nerve fibers, is encased in a tough, fibrous sheath called the epineurium.
Classification of Nerve Fibers
Based on Myelination
- Myelinated Nerve Fibers: These fibers have a myelin sheath and are typically faster in conducting impulses due to saltatory conduction.
- Unmyelinated Nerve Fibers: These fibers lack a myelin sheath and conduct impulses more slowly.
Based on Diameter and Conduction Velocity (Gasser and Erlanger’s classification)
- Group A Fibers: These are large, myelinated fibers with high conduction velocity. They include Aα, Aβ, Aγ, and Aδ fibers.
- Group B Fibers: These are myelinated fibers with intermediate diameter and conduction velocity.
- Group C Fibers: These are small, unmyelinated fibers with slow conduction velocity.
Based on Function
- Afferent (Sensory) Nerve Fibers: Carry sensory information from the body to the CNS.
- Efferent (Motor) Nerve Fibers: Transmit motor commands from the CNS to muscles and glands.
Based on Neurotransmitter Secretion
- Adrenergic Nerve Fibers: Release norepinephrine as a neurotransmitter.
- Cholinergic Nerve Fibers: Release acetylcholine as a neurotransmitter.
Properties of Nerve Fibers
- Excitability: The ability to respond to stimuli and convert them into neural impulses.
- Conductivity: The capability to transmit impulses along the length of the nerve fiber.
- Refractory Period: A period immediately following stimulation during which a nerve or muscle is unresponsive to further stimulation.
- Accommodation: The ability of the nerve fiber to adapt to a slowly increasing stimulus intensity.
- Summation: The additive effect of several small stimuli on a nerve fiber, leading to an action potential if the threshold is reached.
Function of Nerve Fibers
- Transmission of Electrical Impulses: Nerve fibers are responsible for the conduction of electrical impulses, known as action potentials, away from the neuron’s cell body towards other neurons or effector organs.
- Communication: At the axon terminals, the electrical signal triggers the release of neurotransmitters, which cross the synaptic gap and transmit the signal to the next neuron or effector cell.
Electrophysiology
Electrophysiology is the scientific study of the electrical properties of biological cells and tissues. It’s a branch of physiology and biophysics that deals with the flow of ions (ion currents) across cellular membranes and the electrical potentials that arise from these ion movements.
Fundamental Concepts
- Membrane Potential: Cells maintain a voltage difference across their membrane, known as the membrane potential, which is crucial for the function of nerve and muscle cells.
- Ion Channels: Proteins in the cell membrane form channels that allow specific ions to pass through, contributing to the membrane potential and cellular communication.
- Action Potentials: Rapid changes in membrane potential that occur in excitable cells (like neurons and muscle cells) and serve as the basic unit of communication in the nervous system.
Electrophysiological Techniques
- Intracellular Recording: Measures the membrane potential or action potentials within a single cell using a microelectrode.
- Extracellular Recording: Captures the electrical activity of a group of neurons by placing electrodes outside the neuron.
- Patch-Clamp Technique: Allows the study of individual ion channels by isolating a small patch of the cell membrane.
- Electroencephalography (EEG): Records the electrical activity of the brain through electrodes placed on the scalp.
- Electrocardiography (ECG): Measures the electrical activity of the heart to detect and study heart diseases.
Applications of Electrophysiology
- Medical Diagnosis: Used to diagnose and treat conditions like epilepsy, arrhythmias, and other disorders that affect the electrical functioning of the heart and brain.
- Research: Helps in understanding the fundamental mechanisms of cellular function, neural communication, and the basis of neurological diseases.
Action potential
An action potential is a fundamental physiological process that allows neurons to transmit signals over long distances. It’s a complex sequence of events that occurs when a neuron sends information down an axon, away from the cell body.
Resting Membrane Potential
- Before an action potential begins, a neuron is in a resting state where the inside of the neuron is negatively charged relative to the outside.
- This resting membrane potential is typically around -70 millivolts (mV) and is maintained by the sodium-potassium pump and leak channels.
Stages of Action Potential
- Threshold: An action potential is initiated when the neuron receives a signal that causes the resting potential to become less negative, reaching a critical level called the threshold, usually around -55 mV.
- Depolarization: Once the threshold is reached, voltage-gated sodium channels open, allowing Na+ ions to rush into the neuron. This influx of positive ions causes the membrane potential to rise rapidly, resulting in depolarization.
- Overshoot: The membrane potential may reach as high as +30 to +40 mV. At this point, the inside of the neuron is positively charged compared to the outside.
- Repolarization: After the peak is reached, voltage-gated sodium channels close, and voltage-gated potassium channels open. K+ ions flow out of the neuron, which causes the membrane potential to drop back down, becoming more negative.
- Hyperpolarization: The membrane potential temporarily becomes more negative than the resting potential, often reaching around -90 mV. This is due to the potassium channels closing slowly, allowing excess K+ to leave the neuron.
- Return to Resting State: The sodium-potassium pump restores the resting membrane potential by moving 3 Na+ ions out of the neuron and 2 K+ ions into the neuron for each ATP molecule consumed.
Refractory Periods
- Absolute Refractory Period: During this time, the neuron cannot fire another action potential, no matter how strong the stimulus, because the sodium channels are inactivated.
- Relative Refractory Period: The neuron can initiate another action potential, but only if the stimulus is stronger than usual, because the membrane is hyperpolarized.
Propagation of the Action Potential
- The action potential travels down the axon as each adjacent segment of the axon reaches the threshold and depolarizes.
- In myelinated axons, the action potential jumps from one Node of Ranvier to the next, a process known as saltatory conduction, which increases the speed of transmission.
The action potential is an all-or-none event, meaning that once the threshold is reached, the neuron will fire an action potential that is always the same magnitude; it either happens completely or not at all. This process is crucial for neural communication and underlies all neural functions, from sensory perception to muscle control.
Receptors
Receptors in the nervous system are specialized structures that translate various forms of environmental stimuli into electrical signals that the nervous system can understand and process. These receptors are essential for all sensory systems, including vision, hearing, taste, smell, and touch.
Types of Receptors
- Mechanoreceptors: These receptors respond to mechanical pressure or distortion. They are found in the skin, where they detect touch, pressure, vibration, and stretch.
- Thermoreceptors: These detect changes in temperature. There are separate receptors for sensing heat and cold.
- Nociceptors: These are pain receptors that respond to tissue damage or potentially damaging stimuli. They can detect chemical, mechanical, or thermal changes.
- Chemoreceptors: These receptors respond to chemical substances. They are involved in taste and smell and also monitor the chemical composition of the body, such as oxygen and carbon dioxide levels in the blood.
- Photoreceptors: Located in the retina of the eye, these receptors respond to light and enable vision.
- Proprioceptors: These provide information about body position and movement by detecting changes in muscle tension and joint position.
Receptor Structure and Function
- Receptors can be simple nerve endings or complex sensory organs like the eyes or ears.
- They are often categorized based on their location: exteroceptors detect external stimuli, interoceptors detect internal states, and proprioceptors sense body position and movement.
- Receptors convert physical or chemical stimuli into electrical impulses through a process called transduction.
- The region from which a neuron’s receptor can detect stimuli is known as its receptive field.
Signal Transduction Pathways
- When a receptor is activated by a stimulus, it triggers a molecular signal transduction pathway.
- This often involves the opening or closing of ion channels, leading to a change in the receptor’s membrane potential.
- If the change is significant enough, it can generate an action potential that travels along afferent nerve fibers to the central nervous system.
Synaptic Transmission
- The electrical signal from the receptor is often converted into a chemical signal at a synapse, where neurotransmitters are released.
- These neurotransmitters bind to receptors on the postsynaptic cell, which can be another neuron, a muscle cell, or a gland cell.
Adaptation
- Many receptors exhibit adaptation, meaning their response diminishes over time despite continuous stimulation.
- This allows the nervous system to be more sensitive to changes in stimulation rather than constant stimuli.
Receptor Diversity
- The nervous system contains a vast array of receptors, each specialized for different types of stimuli.
- This diversity allows for the fine-tuned perception and response to a complex environment.
Receptors are the initial interface between the nervous system and the world around us, playing a critical role in how we perceive and interact with our environment. They are finely tuned to detect specific types of stimuli and initiate the appropriate response within the nervous system.
Synapse
A synapse is a specialized junction that facilitates communication between neurons, or between neurons and other types of cells, like muscle or gland cells.
Structure of a Synapse
- Presynaptic Neuron: The neuron that sends the signal. It contains synaptic vesicles filled with neurotransmitters.
- Synaptic Cleft: A small gap between the presynaptic and postsynaptic neurons, typically about 20 to 30 nanometers wide.
- Postsynaptic Neuron: The neuron that receives the signal. It has receptors on its surface that are specific to the neurotransmitters released by the presynaptic neuron.
Function of a Synapse
- Signal Transmission: When an action potential reaches the axon terminal of the presynaptic neuron, it triggers the release of neurotransmitters into the synaptic cleft.
- Neurotransmitter Release: The neurotransmitters cross the synaptic cleft and bind to receptors on the postsynaptic neuron.
- Postsynaptic Response: Depending on the type of neurotransmitter and receptor, the binding may excite or inhibit the postsynaptic neuron, affecting the likelihood of it firing an action potential.
Types of Synapses
- Chemical Synapses: Most synapses are chemical. They use neurotransmitters to send signals across the synaptic cleft. The binding of neurotransmitters to receptors can either excite or inhibit the postsynaptic neuron.
- Electrical Synapses: Less common than chemical synapses, electrical synapses allow ions to flow directly between cells through channels known as gap junctions. They enable faster signal transmission and can be bidirectional.
Synaptic Transmission Process
- Arrival of Action Potential: An action potential arrives at the presynaptic axon terminal.
- Vesicle Fusion: Voltage-gated calcium channels open, allowing Ca²⁺ ions to enter the presynaptic neuron, causing synaptic vesicles to fuse with the presynaptic membrane.
- Neurotransmitter Release: Neurotransmitters are released into the synaptic cleft by exocytosis.
- Binding to Receptors: Neurotransmitters bind to receptors on the postsynaptic neuron’s membrane.
- Postsynaptic Potential: This binding causes ion channels on the postsynaptic membrane to open or close, leading to a change in the postsynaptic potential.
- Signal Propagation or Inhibition: If the change in potential is significant enough, it may trigger an action potential in the postsynaptic neuron; otherwise, it may inhibit the neuron from firing.
Synaptic Plasticity
Synapses are not static structures; they can change in strength and efficiency, a property known as synaptic plasticity. This is thought to be the cellular basis for learning and memory.
Neurotransmitter Reuptake and Degradation
After neurotransmitters have bound to receptors, they are typically either taken back into the presynaptic neuron (reuptake) or degraded by enzymes in the synaptic cleft.
Neurotransmitters
Neurotransmitters are the chemical messengers of the nervous system, essential for transmitting signals between neurons or from neurons to other target cells such as muscle cells or glands.
Basic Function
- Neurotransmitters are released from the axon terminal of a neuron into the synaptic cleft, the small gap between neurons.
- They bind to specific receptors on the postsynaptic cell, which can initiate or inhibit an action potential in that cell.
Synthesis and Storage
- Neurotransmitters are synthesized in the neuron, often in the axon terminal or cell body, and stored in vesicles.
- When an action potential reaches the axon terminal, it triggers the release of these stored neurotransmitters into the synaptic cleft.
Release and Reuptake
- The release of neurotransmitters is a calcium-dependent process, where the influx of calcium ions prompts vesicles to fuse with the membrane and release their contents.
- After neurotransmitters have acted on the postsynaptic receptors, they are typically removed from the synaptic cleft either by enzymatic degradation or by reuptake into the presynaptic neuron.
Types of Neurotransmitters
- Acetylcholine: Involved in muscle activation, attention, and arousal.
- Dopamine: Plays a role in reward, motivation, and motor control.
- Serotonin: Affects mood, appetite, and sleep.
- Norepinephrine: Influences attention, response to stress, and heart rate.
- Glutamate: The primary excitatory neurotransmitter in the brain, important for learning and memory.
- GABA (Gamma-Aminobutyric Acid): The main inhibitory neurotransmitter, which helps to regulate neuronal excitability.
Functions in the Body
- Neurotransmitters regulate a vast array of functions, including heart rate, breathing, sleep cycles, digestion, mood, concentration, appetite, and muscle movement.
- They are also involved in cognitive functions such as thoughts, memory, learning, and the response to stress.
Neurotransmitters are integral to the functioning of the nervous system, enabling the complex communication that underlies every thought, feeling, and action. Their study is a key area of neuroscience, with implications for understanding the brain and treating its disorders.
Conclusion
In the grand orchestra of the human body, the nervous system stands as the master conductor, orchestrating a symphony of complex biological processes that underpin our very existence. From the rapid transmission of nerve impulses along the intricate pathways of neurons to the delicate dance of neurotransmitters across synaptic gaps, each element plays a vital role in the harmonious function of this elaborate network.
The nervous system’s receptors serve as the audience, translating the diverse stimuli of our environment into the language of electrical signals. These signals are then meticulously conducted through the neural ensemble, creating the rich tapestry of human experience—from the simple reflex to the profound depths of thought. As we continue to unravel the mysteries of the nervous system, we gain not only a deeper understanding of the biological basis of life but also invaluable insights into the treatment of its disorders.
For more regular updates you can visit our social media accounts,
Instagram: Follow us
Facebook: Follow us
WhatsApp: Join us
Telegram: Join us