Introduction
Conjugated dienes are compounds that contain two adjacent carbon-carbon double bonds. These double bonds are separated by a single carbon atom, which means they’re in a conjugated arrangement. Think of them as the cool kids of the double bond world—they hang out together and share electrons like a tight-knit group. The stabilization of dienes by conjugation is less dramatic than the aromatic stabilization of benzene. Nevertheless, similar resonance and molecular orbital descriptions of conjugation may be written. In this article we will see stability of conjugated dienes, Diel-Alder, electrophilic addition, free radical addition reactions of conjugated dienes and allylic rearrangement.
Stability of conjugated dienes
Conjugated dienes are like the cool kids of the double bond world—they have a certain swagger that sets them apart. So, why are they more stable than their non-conjugated counterparts? Let’s break it down
Delocalization of Charge through Resonance
- Conjugated dienes have alternating single and double bonds, creating a chain of π (pi) electrons. These π electrons can move around freely, thanks to resonance.
- Imagine a little electron dance party where the electrons shimmy between adjacent double bonds. This delocalization spreads out the charge, making the molecule more stable.
- Non-conjugated dienes lack this electron dance floor—they’re just standing awkwardly in one spot.
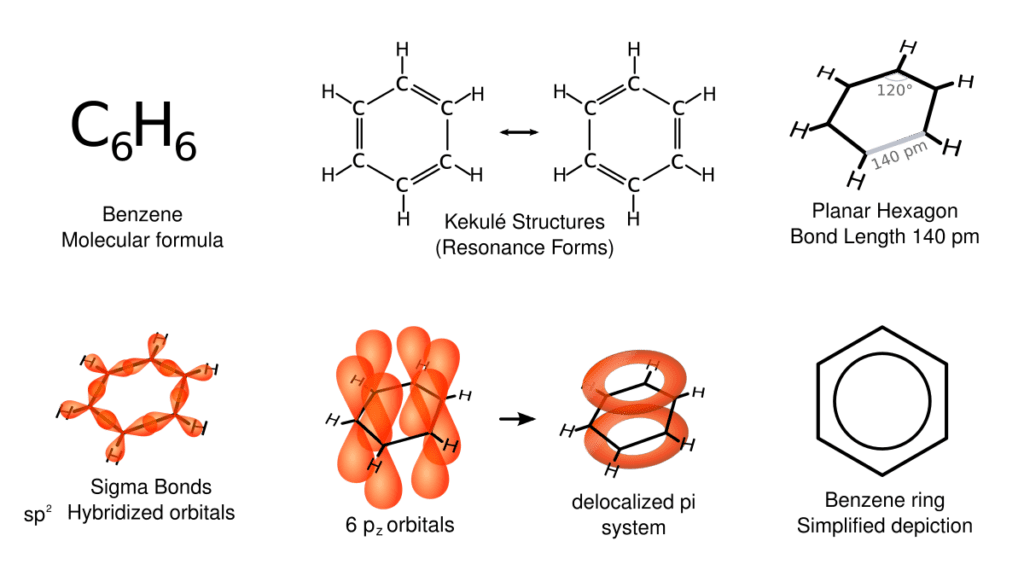
Hybridization Energy
- The hybridization of carbon atoms matters. In conjugated dienes, the carbon atoms involved in the double bonds are sp² hybridized.
- Sp² hybridization allows for better overlap of p orbitals, which leads to stronger π bonds. Stronger bonds = happier molecules.
- Non-conjugated dienes often have sp³ hybridized carbon atoms in between the double bonds. These sp³ carbons are like the party poopers—they disrupt the electron flow.
Heats of Hydrogenation
- Experimentally, we’ve found that conjugated dienes release less heat during hydrogenation (adding hydrogen across the double bonds) than predicted.
- This means their energy content is lower than expected. In other words, they’re more stable than their formula suggests.
Diels-Alder Reaction
The Diels-Alder reaction is like a chemical tango between a conjugated diene (a molecule with alternating double bonds) and a dienophile (an alkene). When they come together, they create a beautiful, six-membered ring—a cyclohexene derivative. Here are the key points:
Formation of Six-Membered Rings
- Imagine the diene and dienophile holding hands and dancing. Their coordinated moves lead to the formation of a new carbon-carbon bond, creating a six-membered ring.
- Otto Diels and Kurt Alder first described this reaction in 1928, and for their elegant chemistry moves, they snagged the Nobel Prize in Chemistry in 1950.
Concerted Mechanism
- The Diels-Alder reaction is a pericyclic reaction, meaning it happens all at once—no intermediates, no waiting around. The reactants waltz directly into a cyclic transition state.
- It’s like synchronized swimming for molecules: the diene’s π electrons (the swirly dance partners) and the dienophile’s π electrons (the other swirly dancers) join hands in perfect harmony.
Orbital Symmetry Magic
- The reaction’s success depends on orbital symmetry. Specifically, it’s a [4+2] cycloaddition with a Woodward-Hoffmann symbol of [π^4s + π^2s].
- In plain English: The diene’s highest occupied molecular orbital (HOMO) interacts with the dienophile’s lowest unoccupied molecular orbital (LUMO). They fit together like puzzle pieces, leading to a smooth reaction.
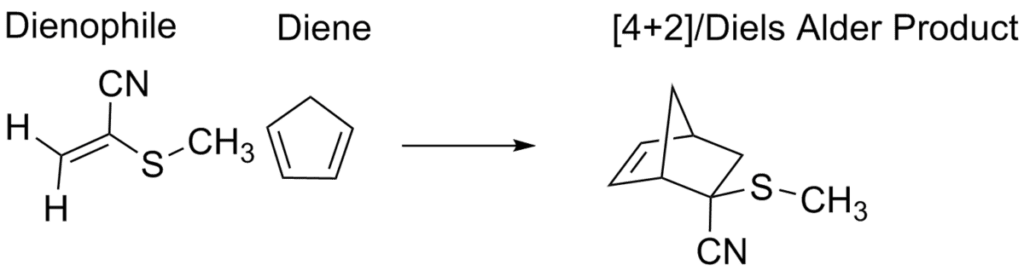
Hetero-Diels-Alder
Sometimes, the dance partners involve heteroatoms (like carbonyls or imines). When that happens, we call it the hetero-Diels-Alder reaction. They still form rings, but with a twist!
Retro-Diels-Alder
- Picture this: After the dance, sometimes the molecules decide to go back to their original positions. This reverse reaction is the retro-Diels-Alder.
- But don’t worry, it’s not a breakup; it’s just a temporary separation.
Electrophilic Addition to Conjugated Dienes
Now, let’s talk about electrophilic addition to these cool conjugated dienes:
1,2-Addition (Markovnikov Style)
- When you add one equivalent of an electrophile (like HX) to a conjugated diene, it’s like giving them a high-five. The electrophile bonds to one of the end carbon atoms (carbon #1), forming an allylic carbocation intermediate.
- These allylic carbocations are like the popular kids—they’re stabilized by resonance. 🎶
1,4-Addition (The Unexpected Twist)
- But wait, there’s more! During electrophilic addition, we get an unexpected product too. The electrophile bonds at the terminal carbon atoms, and the remaining double bond shifts.
- This 1,4-addition product is also influenced by resonance and charge delocalization.
Kinetic vs. Thermodynamic Control
- Sometimes, the reaction gives us a mixture of two products—the kinetic product (formed faster) and the thermodynamic product (more stable).
- The ratio depends on reaction conditions and temperature. It’s like choosing between a spontaneous dance move (kinetic) and a well-practiced routine (thermodynamic).
Free Radical Addition Reactions of Conjugated Dienes
Conjugated dienes—those molecules with alternating double bonds—have a flair for the dramatic. Not only do they engage in their usual chemical reactions, but they also love to tango with free radicals. Here’s the scoop:
1,2- and 1,4-Addition Products
- When you add a free radical (like H•) to a conjugated diene, interesting things happen. The most common products are 1,2-addition and 1,4-addition products.
- 1,2-Addition (Markovnikov Style): The free radical bonds to one of the end carbon atoms (#1), creating an allylic cation intermediate. These cations are like the cool kids—they’re stabilized by charge delocalization.
- 1,4-Addition (The Unexpected Twist): But wait, there’s more! Sometimes the double bond shifts during the reaction. The terminal carbon atoms get involved, and voilà—the 1,4-addition product emerges.
Equilibrium Control and Product Ratios
- In reactions where both 1,2 and 1,4 additions occur simultaneously, the product ratio isn’t fixed. It depends on factors like temperature, solvent, and reaction time.
- The reversible nature of carbocation formation means that the equilibrium product ratio doesn’t necessarily match the initial attack ratio by the free radical.
- Think of it as a dance floor where both 1,2 and 1,4 moves are happening, and the DJ (temperature and conditions) decides the playlist.
Radical-Chain Mechanisms
- Conjugated dienes love a good radical-chain party. The 1,4-adduct is usually the star of the show.
- For example, hydrogen bromide radical addition to 1,3-butadiene gives us l-bromo-2-butene. It’s like the diene and the bromine radical decided to do the cha-cha together.
Allylic Rearrangement
Now, let’s talk about allylic rearrangement. Imagine a double bond doing the electric slide—it shifts to an adjacent pair of carbon atoms. Here’s the lowdown
Why Does It Happen?
- Allylic shifts occur because the transition state resembles an allyl intermediate. These intermediates are like the backstage crew—they make the magic happen.
- Allylic rearrangements can occur during both nucleophilic and electrophilic substitutions.
Nucleophilic Allylic Shifts
- When a nucleophile attacks near the double bond, the pi electrons shimmy over, and the double bond relocates.
- Steric hindrance or destabilizing substituents can trigger allylic shifts. It’s like the double bond saying, “I need more space!”
Electrophilic Allylic Shifts
- Electrophiles can also join the dance. For instance, in the Prins reaction, the carbonyl group in benzaldehyde gets activated by diboronic acid.
- They for a single regioisomer and stereoisomer—like a perfectly choreographed routine.
Flip-Flopping Double Bonds
- Sometimes, repeated allylic shifts flip-flop a double bond between two possible locations. It’s like the double bond is indecisive about its dance partner.
- Picture an aziridine doing the twist with methyllithium—acetylene gets lost in the process, and the double bond changes partners.
Summary
We explored the captivating world of conjugated dienes and their chemistry dance moves. We kicked off with the stability of conjugated dienes, discussing resonance, hybridization, and heats of hydrogenation. Then, we twirled into the Diels-Alder reaction, where dienes and dienophiles waltz to form six-membered rings. Electrophilic addition joined the party, with 1,2 and 1,4 products showing off their moves.
For more regular updates you can visit our social media accounts,
Instagram: Follow us
Facebook: Follow us
WhatsApp: Join us
Telegram: Join us